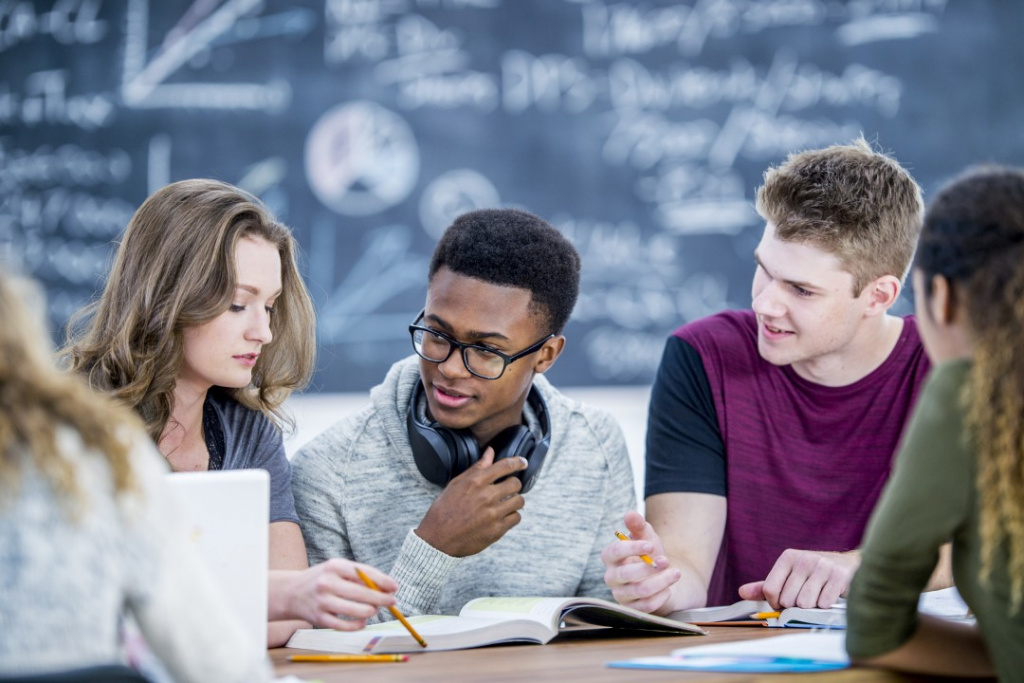
Background
We teach at a sixth form college in Cambridge with around 2,600 students from all over the East of England. We have a chemistry cohort of around 80 students per year, whose prior attainment is lower than the national average.
Having taught A-Level Chemistry (UK-based qualification for 16-to-19-year-old students) for more than 40 years collectively, we have found that students frequently have significant misconceptions relating to aspects of polymer chemistry. We have also found that thinking carefully about the sequence in which ideas are taught can aid students in appreciating the concept of ‘scale’ regarding the relative size of particles and facilitate understanding and progress.
We have already discovered that, for some of our students, scale is a ‘threshold concept’ – an idea that is initially challenging to understand but, once grasped, permanently transforms a student’s thinking (Colebrooke, S.; Thorn, Z., 2024). It is analogous to passing through a portal or gateway in that their understanding on the other side of the threshold is completely changed (Meyer, J.F.H.; Land, R., (Eds.), 2006).
To implement these discoveries practically, we began to teach bonding and structure in order of increasing scale, completely re-sequencing our previous approach (Thorn, Z.; Colebrooke, S., 2024). For example, in our new sequence, students meet the ‘mole concept’ for the first time with samples consisting of single atoms. Later they study moles of molecules, then later still moles of giant structures.
The transformation of the sequence of our A-level teaching started as part of a ‘Close-to-Research Professional Development’ project undertaken with Anglia Ruskin University (Kirkman, P; Caws, D; Childs, C; Colebrooke, S.; Issot, M.; Parkin, J.; Thorn, Z., 2023) (Kirkman, P.; Conolly, S.; Caws, D.; Bowser-Angemann, J.; Durham, H.; Fossey, R.; Gray, L.; Parkin, J.; Platt, R., 2023).
We now appreciate how significant ‘scale’ is elsewhere in the A-level chemistry course. We aim to demonstrate how recognition of scale as an important threshold concept can be applied to teaching aspects of polymer chemistry and structural biochemistry, by developing suitable teaching sequences and designing questions that make the significance of scale explicit.
Sequencing in action
At the time we teach these concepts, students have already encountered simple molecular substances and giant structures (interpreted as large three-dimensional arrays). They have not been introduced to polymers at A-level, though may well have studied polymers to some extent at GCSE level. Polymers are simple molecular substances, although they are substantially larger than the molecules students have commonly studied earlier in the course.
We have identified common misconceptions that students often hold about polymers, such as thinking that polymers are giant structures, as they contain many hundreds of atoms. They also often have difficulty drawing a repeating unit from monomer structures or vice versa as they are unable to see where parts of the molecules ‘fit’ or even what a repeating structure actually is.
We have re-sequenced our teaching order to give a clearer sense of the scale levels involved. The new and previous sequences are compared in the diagram below. It is not straightforward to re-sequence entirely in order of scale due to the complexity of the structures and the context involved, but the new order is more logical by scale.
One thing the sequence achieves is to reduce the number of times students change scale from seven changes between SM (simple molecular) and LSM (large simple molecular) in our original sequence to only three changes in the new sequence. In fact, the scale only changes when there is a significant increase in the complexity of monomers forming polymers – when amino acids are introduced – and other aspects of their structure need to be considered first (such as optical isomerism).
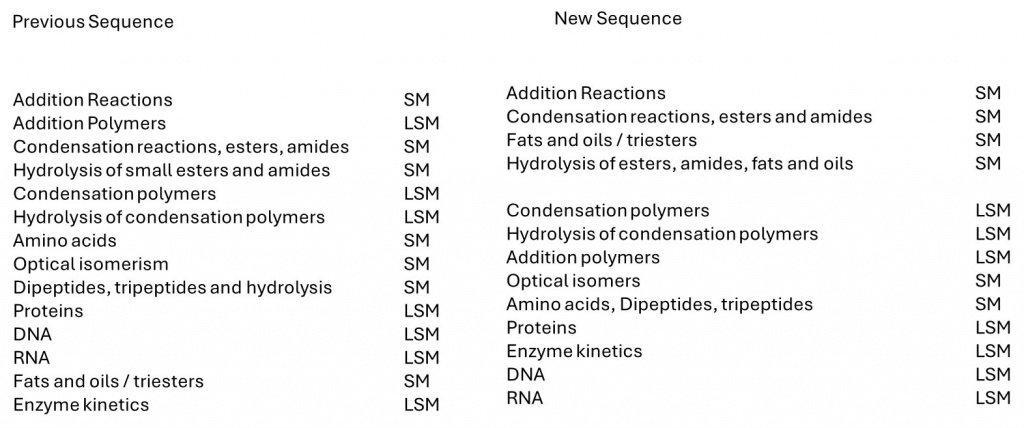
The sequence shows that condensation and hydrolysis reactions are first introduced for small molecules with single functional groups (FGs), then for fats and oils with multiple FGs and higher molecular masses. Learners are then introduced to larger-scale size polymers with many FGs at regular intervals, finally revisiting hydrolysis for a third time.
The biochemistry topic adds an increase in scale together with a further layer of complexity, with different building blocks and chirality; first small molecules, then proteins. These biological polymers are more complex than nylons and polyesters, as they fold into a specific 3D shape rather than just having random intermolecular forces between chains. A summary is shown in the diagram below.
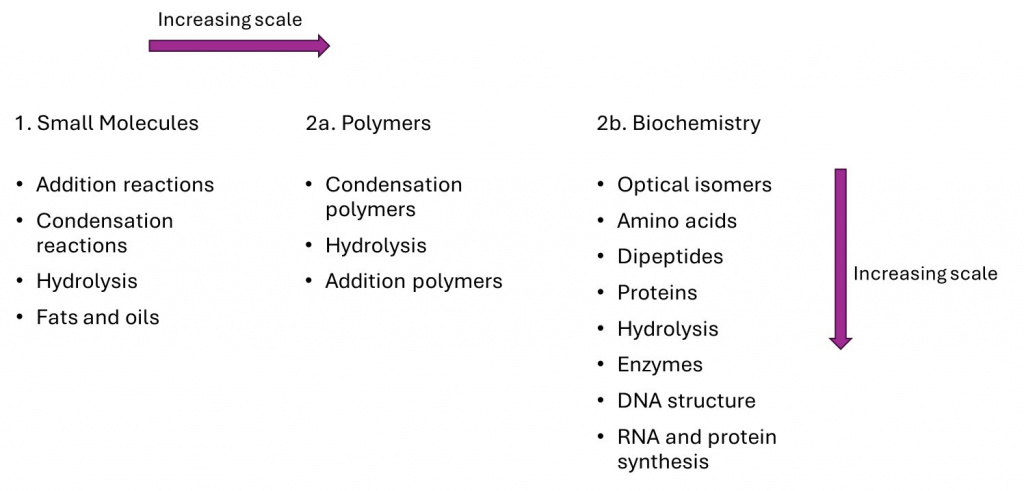
Our new teaching sequence introduces concepts in order of increasing molecular size and complexity. Reactions of organic molecules with single functional groups are introduced first: alkenes, carboxylic acids and amines. Then students learn about condensation reactions between single FGs in two different reactants to create esters or amides. Finally, in this section, the learning experience is extended to fats and oils – triesters with multiple FGs – made from more complex carboxylic acids and triols.
At this stage, we move to the polymer scale, starting with polyesters as these functional groups are most familiar – the only change being the number of condensation reactions each molecule can undergo: two in order to form a chain. Here we also introduce the repeat unit concept (more on this below). Secondly, we add polyamides, changing the functional group which forms but not the reaction type. Thirdly, we introduce addition polymers to see the extension of polymers to those formed by a different type of reaction. We have found that students tend to find addition polymers more complex, hence these are studied after condensation polymers.
Finally, we extend all these ideas into the context of biochemistry. Proteins have more complicated monomers (amino acids, with two different FGs in addition to side chains of various structures) and they can be connected by condensation reactions in any combination. Students start by combining two or three amino acids to learn about the structures and naming of peptides, then look at polypeptides.
The picture is further complicated by the polymer chain adopting a global tertiary structure, with different types of interactions between parts of the same chain. Other biomolecules – DNA and RNA – are considered last as they are made from even more complex monomers (nucleotides) which are themselves formed via condensation involving three different, smaller molecules.
Two additional features of our teaching have been modified to try to facilitate student understanding of this topic:
Reference to Johnstone’s triangle
One of the challenges associated with teaching and learning in chemistry is the frequent changes in the type of representation that students need to become familiar with and switch between. This difficulty was described by Johnstone (Johnstone, 2010) in the form of a triangle, the corners of which signify the different levels at which students need to think: macroscopic, sub-microscopic and representation. To progress in any one area of chemistry, students frequently need to switch repeatedly between all three levels. This diagram shows aspects of polymer chemistry transposed onto Johnstone’s triangle.
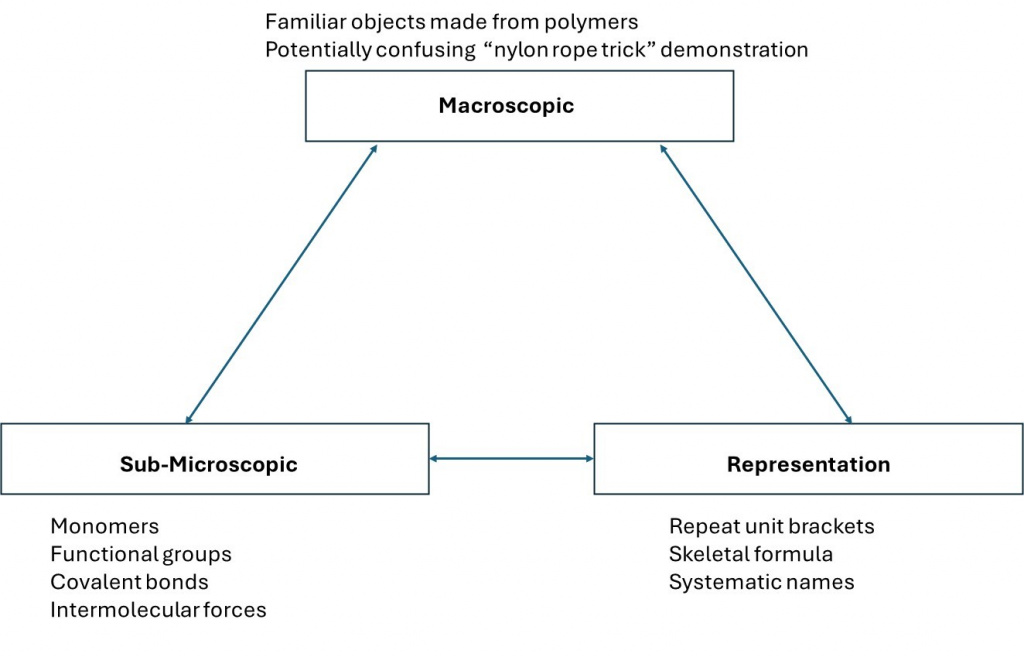
We made several changes to our teaching strategies to help students with the concept of ‘polymer repeat units’, which from past experience we know many students find a challenging concept. The repeat unit is a way of representing a very long repetitive portion of the structure of a polymer chain and is usually represented as shown in the example in the diagram below.
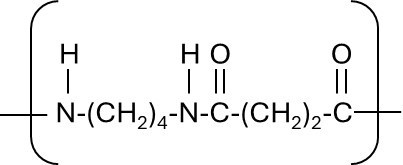
The bracket encloses the smallest portion of the polymer chain that repeats multiple times along the much longer polymer chain. This therefore connects the bottom two corners of Johnstone’s triangle – the sub-microscopic bonding of atoms into a chain and the representation of a long chain with enough information to understand the whole structure. When linking to the top of the triangle, demonstrations such as the well-known “Nylon Rope Trick” must be used carefully to avoid giving the impression that an individual polymer chain is large enough to be visible to the naked eye.
We found it possible to strengthen the student link between the sub-microscopic and representation by initially reminding students of situations in which they have met repeating sequences in the past; for example, in primary-age maths, with simple geometric shapes. These are used to introduce students to the square bracket notation, before asking students to generate a repeating sequence from a square bracket notation.
Having established the representation of square brackets, we then introduced sub-microscopic structures and essentially repeated the previous questions – identify the repeat unit, then draw polymer structures given the square bracket repeat unit.
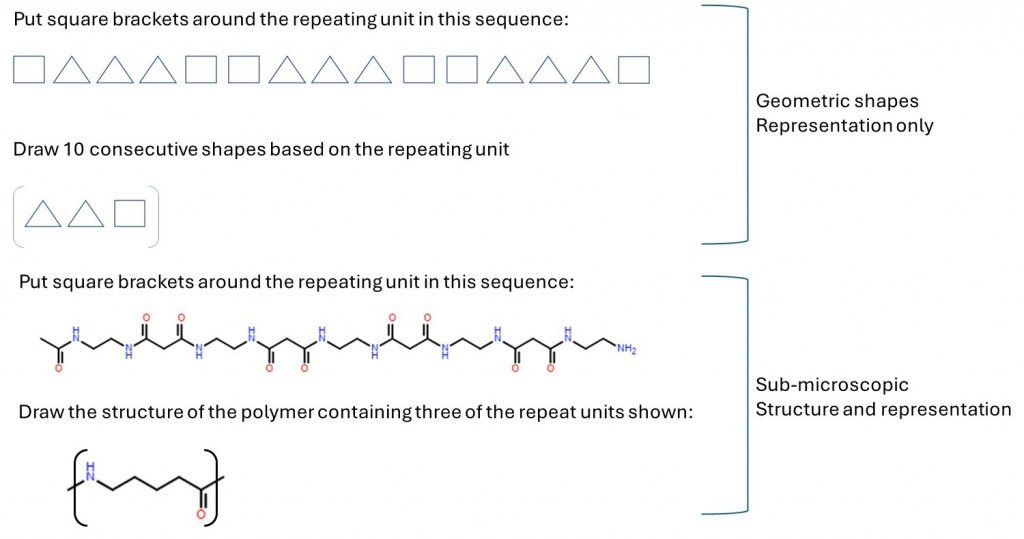
Another tactic was utilised with polymer hydrolysis; printing long sections of different polymer chains and getting students to physically cut these up into smaller pieces at positions where hydrolysis occurs.
Frequent calculations and other activities to emphasise and compare scales
Finally, we have consistently driven students to reconsider aspects of scale in this topic by making numerous explicit references to the relative size of molecules involved. We have found the following exercise ideas useful:
- Calculation of the molecular formulae and relative molecular masses of fat molecules. These are not difficult calculations for students at this level, and we have not taken the time to do these previously. However, as the values obtained are much larger than those students have been used to calculating, it reinforces the relatively large size of a fat molecule compared to simple esters containing 3-10 carbon atoms.
- Calculation of the number of monomers needed to generate a polymer chain of a given relative molecular mass. This calculation reinforces the concept of polymers being composed of numerous smaller molecules in a chain. Calculations can be done in either direction. Also useful is the calculation of the mass of a polymer chain when given the number of repeat units it contains.
- Calculation of the total number of atoms in a polymer chain based on the molecular formula of a repeat unit.
- Calculation of the physical dimensions of an enzyme/protein molecule – useful for comparing to the dimensions of a much smaller substrate, but also to reinforce the idea that proteins are not giant structures.
- We have based questions on the molecular formulae and formula masses of some protein molecules and how many amino acid residues must be present. For example: ‘The formula of the protein haemoglobin is C2932H4724N828O840S8Fe4. How many atoms does it contain? What is the maximum number of cysteine residues in the protein?’ These questions are unlikely to be asked in exam papers, but they force students to think again about the composition of a protein.
- Demonstrating examples of structural formulae at locations in protein chains, identifying chiral carbons or particular amino acid residues in different sections.
Outcomes
We have found that our students have been thinking more carefully about polymer and biochemical structures. Some of the misconceptions have been less prevalent, though this is hard to quantify. Students now have a better understanding of how monomers join and why the molecule must have the capacity to bond with two other monomers.
In addition, we have been asked many more questions, such as ‘What is at the end of a polymer chain?’ or ‘What is the mechanism for making an addition polymer, because it must be different from simple electrophilic addition?’ We have overheard some students having ‘lightbulb’ moments: ‘Oh, so that’s what happens in hydrolysis.’ As teachers, we have also rethought much of our terminology and strategy and thought much more about representation to help students.
Conclusion
Careful thinking about the sequence in which ideas are introduced to students – particularly scale and size – can help build up their understanding of polymers and biochemistry and link new ideas to molecules they are already familiar with. It can help them overcome particular barriers to learning and grasp new threshold concepts. We have found that it is worth creating a bespoke learning pathway for our students by sequencing ideas from across the exam board specification and not simply teaching in ‘textbook’ order.
We have also begun to think much more carefully about how to sequence within one lesson/learning episode, as illustrated by the work with polymer repeat units. Although we have described a very specific context, we anticipate that thinking carefully about scale could help students in many other areas of chemistry and science.
Zoë and Simon both completed PhDs in chemistry before training as teachers. They have over 40 years of combined experience, mostly teaching A-level chemistry, and have worked together at Long Road Sixth Form College in Cambridge UK for the majority of that time. The challenges associated with helping students online during the pandemic proved to be a turning point in their approach to teaching chemistry; they have begun to identify many conceptual barriers for their students and engaged with research to re-order their teaching sequences. They enjoy designing new resources to improve student understanding and help them overcome key thresholds.
References
Colebrooke, S.; Thorn, Z., 2024. Introducing A level Chemistry Concepts in Order of Increasing Scale. School Science Review: In Depth (submitted).
Johnstone, A., 2010. You Can’t Get There From Here. Journal of Chemical Education, 87(1), pp. 22-29.
Kirkman, P.; Conolly, S.; Caws, D.; Bowser-Angemann, J.; Durham, H.; Fossey, R.; Gray, L.; Parkin, J.; Platt, R., 2023. Contextualising Professional Enquiry: “Outsiders” constructing “close-to-research practice”. Symposium presented to BERA 2023. Aston University, s.n.
Kirkman, P; Caws, D; Childs, C; Colebrooke, S.; Issot, M.; Parkin, J.; Thorn, Z., 2023. What does it mean to be research-informed?. Impact, Volume 19.
Meyer, J.F.H.; Land, R., (Eds.), 2006. Overcoming Barriers to Student Understanding, Threshold Concepts and Troublesome Knowledge. 1st Ed. ed. Oxford, New York: Routledge.
Thorn, Z.; Colebrooke, S., 2024. Strategies to Explore and Overcome Misconceptions in A level Chemistry. School Science Review: In Practice (submitted).
Register for free
No Credit Card required
- Register for free
- Free TeachingTimes Report every month